WQI publications
WQI Publications
Finding some wiggle room in semiconductor quantum computers
A new housing for quantum semiconductors upends conventional wisdom, achieving more perfect computations thanks to its less-than-perfect design.
Read the full article at: https://news.wisc.edu/finding-some-wiggle-room-in-semiconductor-quantum-computers/UW–Madison, industry partners run quantum algorithm on neutral atom quantum computer for the first time
A university-industry collaboration has successfully run a quantum algorithm on a type of quantum computer known as a cold atom quantum computer for the first time. The achievement by the team of scientists from the University of Wisconsin–Madison, ColdQuanta and Riverlane brings quantum computing one step closer to being used in real-world applications.
The work out of Mark Saffman’s group was published in Nature on April 20.
Read the press release tipsheet
Ultraprecise atomic clock poised for new physics discoveries
Wisconsin Quantum Institute physicists have made one of the highest performance atomic clocks ever, they announced Feb. 16 in the journal Nature.
Their instrument, known as an optical lattice atomic clock, can measure differences in time to a precision equivalent to losing just one second every 300 billion years and is the first example of a “multiplexed” optical clock, where six separate clocks can exist in the same environment. Its design allows the team to test ways to search for gravitational waves, attempt to detect dark matter, and discover new physics with clocks.
“Optical lattice clocks are already the best clocks in the world, and here we get this level of performance that no one has seen before,” says Shimon Kolkowitz, a UW–Madison physics professor with WQI and senior author of the study. “We’re working to both improve their performance and to develop emerging applications that are enabled by this improved performance.”
Atomic clocks are so precise because they take advantage of a fundamental property of atoms: when an electron changes energy levels, it absorbs or emits light with a frequency that is identical for all atoms of a particular element. Optical atomic clocks keep time by using a laser that is tuned to precisely match this frequency, and they require some of the world’s most sophisticated lasers to keep accurate time.
By comparison, Kolkowitz’s group has “a relatively lousy laser,” he says, so they knew that any clock they built would not be the most accurate or precise on its own. But they also knew that many downstream applications of optical clocks will require portable, commercially available lasers like theirs. Designing a clock that could use average lasers would be a boon.
Correlated errors in quantum computers emphasize need for design changes
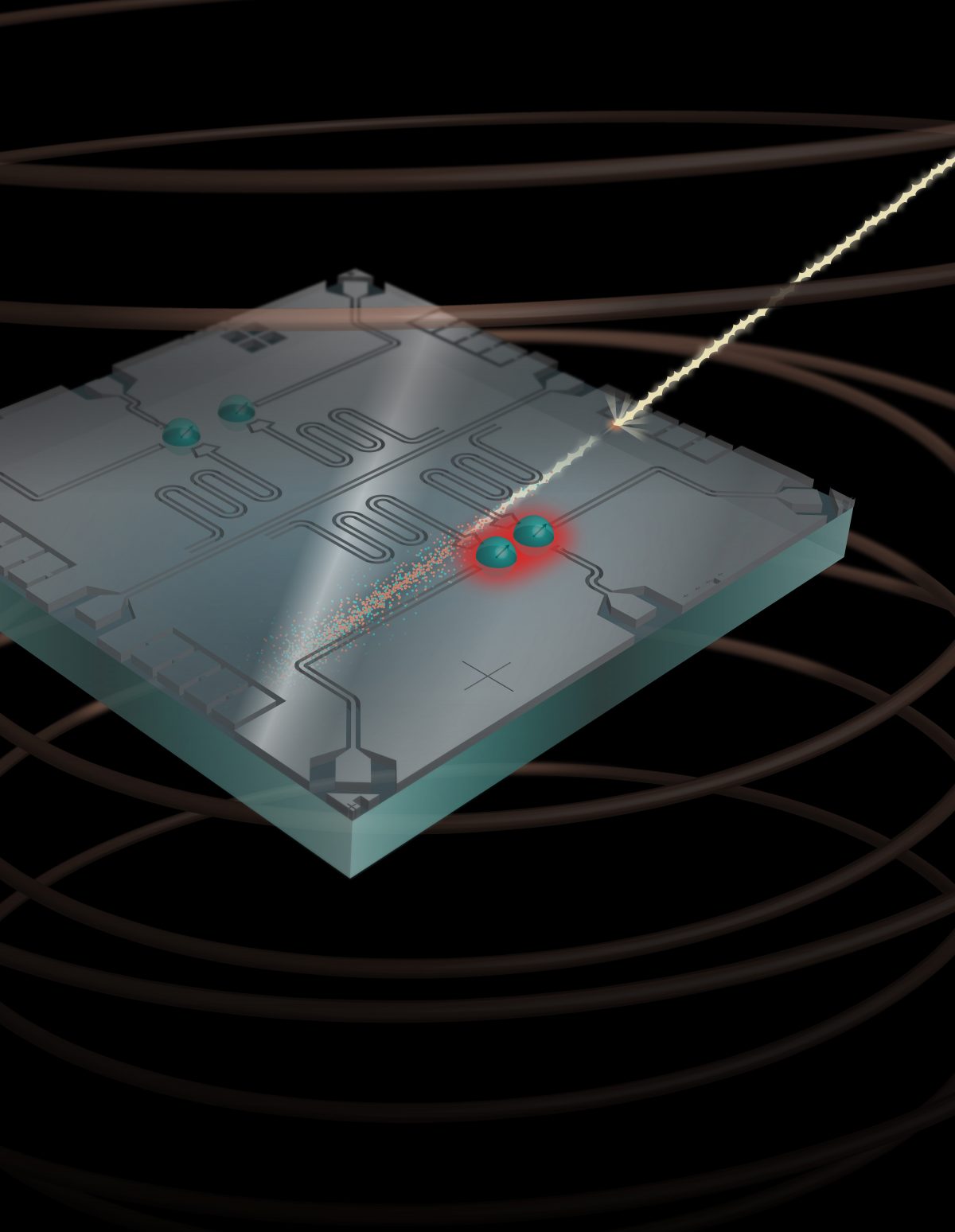
Quantum computers could outperform classical computers at many tasks, but only if the errors that are an inevitable part of computational tasks are isolated rather than widespread events.
Now, researchers at the University of Wisconsin–Madison have found evidence that errors are correlated across an entire superconducting quantum computing chip — highlighting a problem that must be acknowledged and addressed in the quest for fault-tolerant quantum computers.
The researchers report their findings in a study published June 16 in the journal Nature, Importantly, their work also points to mitigation strategies.
“I think people have been approaching the problem of error correction in an overly optimistic way, blindly making the assumption that errors are not correlated,” says UW–Madison physics Professor Robert McDermott, member of the Wisconsin Quantum Institute and senior author of the study. “Our experiments show absolutely that errors are correlated, but as we identify problems and develop a deep physical understanding, we’re going to find ways to work around them.”
Read the full story at https://news.wisc.edu/correlated-errors-in-quantum-computers-emphasize-need-for-design-changes/
MSPQC student Jacques Van Damme publishes first-author paper with WQI faculty
Congrats to Jacques Van Damme, a member of the first class of MSPQC students who graduated this past August, on his first-author publication! The study, published in Physical Review A, is titled, “Impacts of random filling on spin squeezing via Rydberg dressing in optical clocks.” Co-authors include WQI faculty members Mark Saffman, Maxim Vavilov, and senior author Shimon Kolkowitz. | Link to the PRA publication
Surprising communication between atoms could improve quantum computing
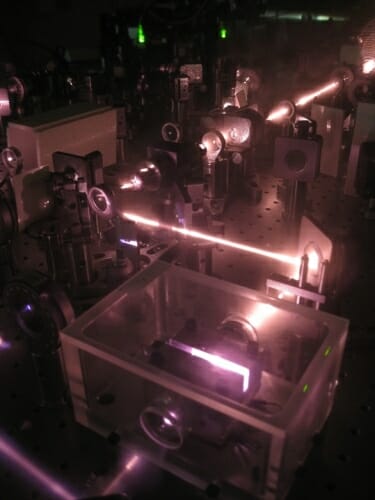
A group of University of Wisconsin–Madison physicists has identified conditions under which relatively distant atoms communicate with each other in ways that had previously only been seen in atoms closer together — a development that could have applications to quantum computing.
The physicists’ findings, published Oct. 14 in the journal Physical Review A, open up new prospects for generating entangled atoms, the term given to atoms that share information at large distances, which are important for quantum communications and the development of quantum computers.
“Building a quantum computer is very tough, so one approach is that you build smaller modules that can talk to each other,” says Deniz Yavuz, a UW–Madison physics professor and senior author of the study. “This effect we’re seeing could be used to increase the communication between these modules.”
The scenario at hand depends on the interplay between light and the electrons that orbit atoms. An electron that has been hit with a photon of light can be excited to a higher energy state. But electrons loathe excess energy, so they quickly shed it by emitting a photon in a process known as decay. The photons atoms release have less energy than the ones that boosted the electron up — the same phenomenon that causes some chemicals to fluoresce, or some jellyfish to have a green-glowing ring.
“Now, the problem gets very interesting if you have more than one atom,” says Yavuz. “The presence of other atoms modifies the decay of each atom; they talk to each other.”
New method measures temperature within 3D objects
University of Wisconsin–Madison engineers have made it possible to remotely determine the temperature beneath the surface of certain materials using a new technique they call depth thermography. The method may be useful in applications where traditional temperature probes won’t work, like monitoring semiconductor performance or next-generation nuclear reactors.
Many temperature sensors measure thermal radiation, most of which is in the infrared spectrum, coming off the surface of an object. The hotter the object, the more radiation it emits, which is the basis for gadgets like thermal imaging cameras.
Depth thermography, however, goes beyond the surface and works with a certain class of materials that are partially transparent to infrared radiation.
“We can measure the spectrum of thermal radiation emitted from the object and use a sophisticated algorithm to infer the temperature not just on the surface, but also underneath the surface, tens to hundreds of microns in,” says Mikhail Kats, a UW–Madison professor of electrical and computer engineering. “We’re able to do that precisely and accurately, at least in some instances.”
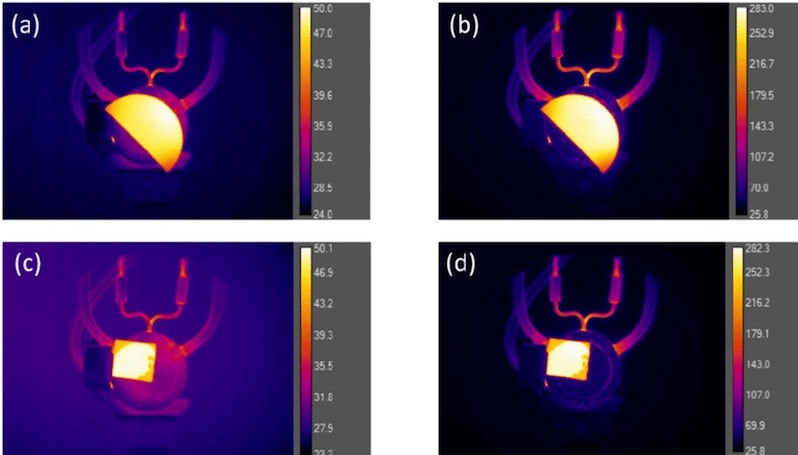
Quantum computing with semiconductor spins
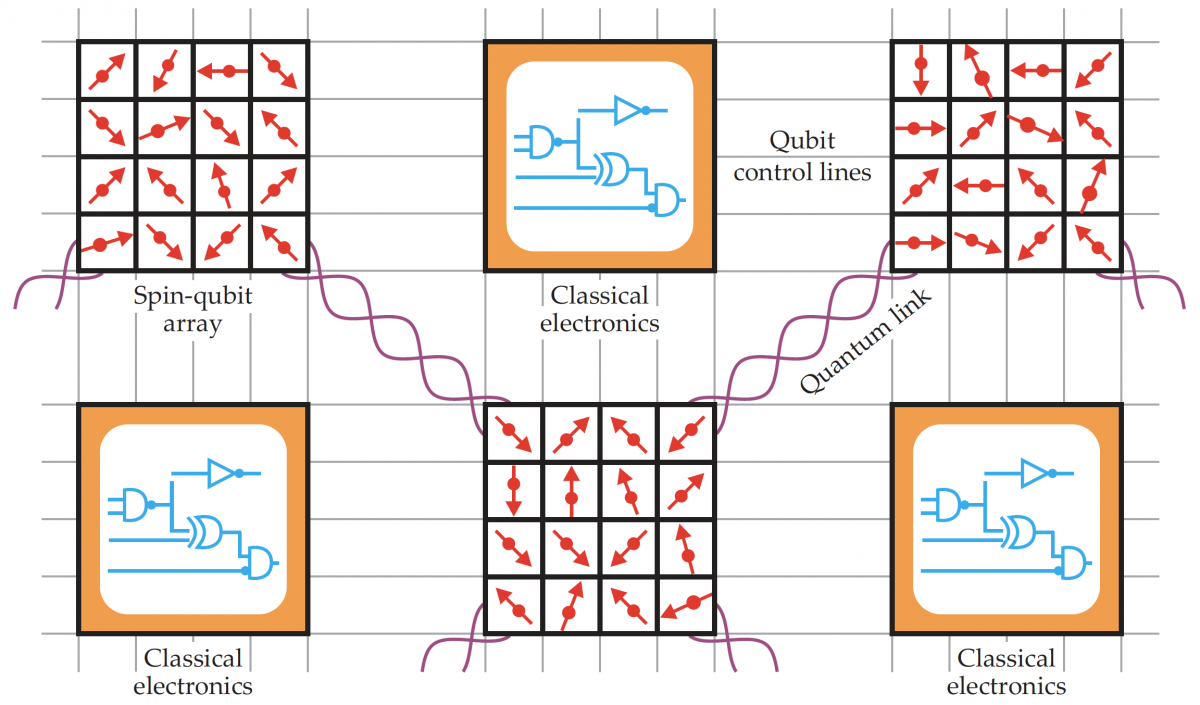
WQI’s Mark Eriksson co-authored a piece, “Quantum computing with semiconductor spins,” in the August 2019 issue of Physics Today.
The full article is available at Physics Today’s website.
Measurement of a superconducting qubit with a microwave photon counter
The superconducting qubit group at WQI introduced an approach to measurement based on a microwave photon counter demonstrating raw single-shot measurement fidelity of 92% [Science, 361, 1239 (2018)]. This scheme provides access to the classical outcome of projective quantum measurement at the millikelvin stage and could form the basis for a scalable quantum-to-classical interface, see this article for broader perspective on this technology.
Read the full article at: http://science.sciencemag.org/content/361/6408/1239