The Eriksson group, including first author, grad student Sam Neyens, recently published the paper “Measurements of Capacitive Coupling Within a Quadruple-Quantum-Dot Array” in Physical Review Applied. In it, they present measurements of the capacitive coupling energy and the interdot capacitances in a linear quadruple-quantum-dot array in undoped Si/SiGe. They provide a model for the capacitive coupling energy based on the electrostatics of a network of charge nodes joined by capacitors, which shows how the coupling energy should depend on inter-double-dot and intra-double-dot capacitances in the network, and find that the expected trends agree well with the measurements of coupling energy.
WQI News
WQI Director wins WARF Innovation Award
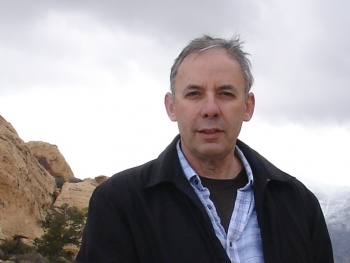
WQI Director and professor of physics, Mark Saffman, won a 2019 WARF Innovation Award.
Saffman has developed simplified optical hardware for quantum computing. His technology, recently licensed to ColdQuanta Inc., improves an apparatus for particle trapping, which will reduce the cost and complexity of next-generation quantum computing devices.
An independent panel of judges selected the Innovation Award winners from a field of six finalists drawn from among approximately 350 invention disclosures submitted to WARF over the past 12 months. The winning inventions each receive an award of $10,000, with the funds going to the UW–Madison inventors named on the breakthroughs.
Read the full story about Saffman’s award and WARF Innovation Day here.
Shimon Kolkowitz earns 2019 Packard Fellowship
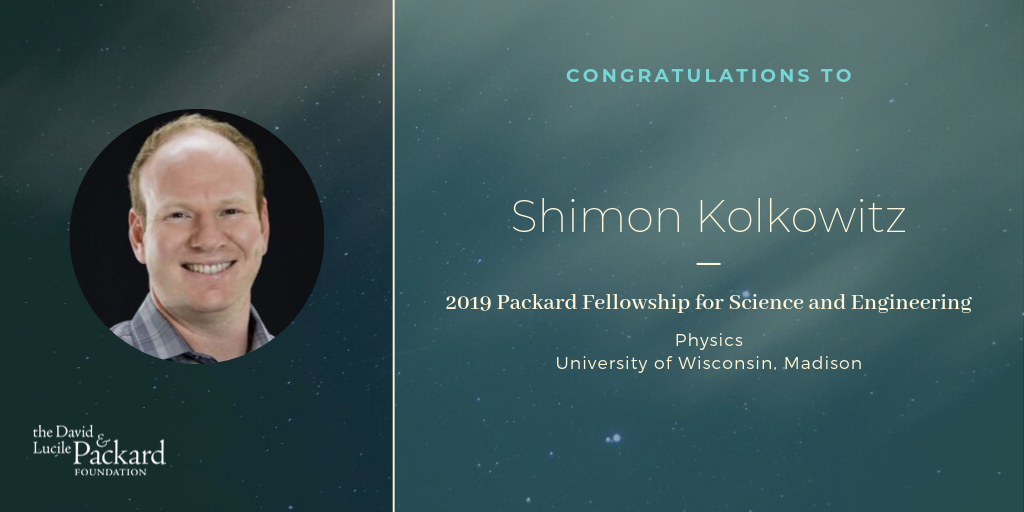
Shimon Kolkowitz, a University of Wisconsin–Madison assistant professor of physics, has been selected as one of 22 members of the 2019 class of Packard Fellows for Science and Engineering.
The fellowship, awarded to early-career scientists from across the U.S., provides $875,000 of funding over five years. Kolkowitz will use the funds to develop his research program in ultra-precise atomic clocks, which he will use to investigate such fundamental aspects of physics as the relationship between quantum mechanics and gravity and the nature of dark matter.
Chicago Quantum Summit to gather international experts
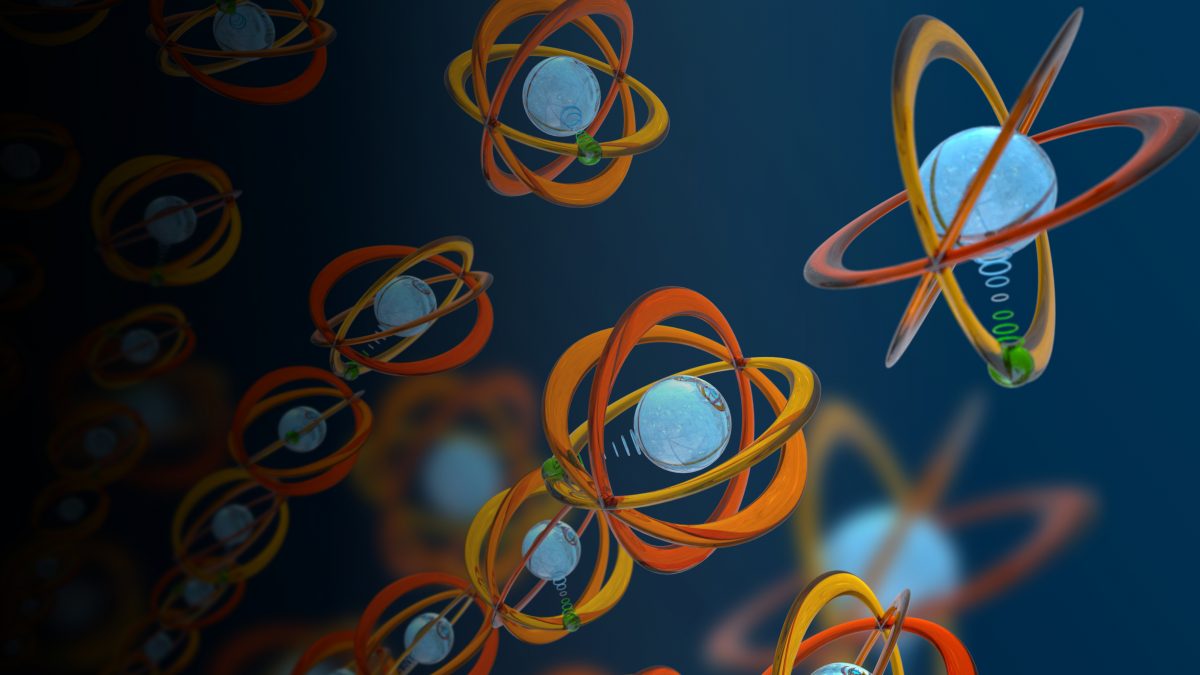
Top experts in quantum technology from around the globe — including experts from the Wisconsin Quantum Institute — will gather at the University of Chicago on Oct. 25 to discuss the future of quantum information science and strategies to build a quantum workforce.
The second annual Chicago Quantum Summit, hosted by the Chicago Quantum Exchange, will engage scientific and government leaders and the industries that will drive the applications of emerging quantum information science.
Response of a quantum disordered spin system to a local periodic drive
A new publication from the Vavilov group, from A. Barış Özgüler, Canran Xu, is on arXiv! Read the full paper on the arXiv site.
WQI’s Wu awarded grant to advance quantum computing machine learning
The US Department of Energy recently announced the funding of another set of quantum science-driven research proposals, including that of Sau Lan Wu, Enrico Fermi professor of physics and Vilas Professor at the University of Wisconsin–Madison. With the funding, Wu and her collaborators seek to tap into the power of quantum computing to analyze the wealth of data generated by high energy physics experiments.
The title of Wu’s DOE approved project is: “Application of Quantum Machine Learning to High Energy Physics Analysis at LHC using IBM Quantum Computer Simulators and IBM Quantum Computer Hardware”.
Wu, a member of the Chicago Quantum Exchange (CQE) and Wisconsin Quantum Institute at UW–Madison who conducts her research at the Large Hadron Collider (LHC) at CERN in Geneva, Switzerland, was one of only six university-based investigators – those outside of National Labs – to be awarded the DOE quantum funds for particle physicists.
“The ambitious HL-LHC program will require enormous computing resources in the next two decades,” says Wu. “A burning question is whether quantum computers can solve the ever-growing demand for computing resources, and our goal here is to explore and to demonstrate that quantum computing can be the new paradigm.”
Wu’s research program has one major goal: to discover new physics. This goal requires the identification of rare signals in immense backgrounds. Wu’s group has pioneered the use of machine learning algorithms on standard computers to move quickly and accurately to extract the physics signal. Sifting through the data to find meaningful and incredibly rare signals takes inordinate computing power at LHC.
“However, because of the rapidly increasing volume of data in the future HL- LHC program, our current machine learning algorithms just don’t have enough computing power to conduct complex analyses,” Wu says. “We believe that applying quantum machine learning methods may well be a new direction to go.”
For her DOE-funded project, Wu works with collaborators in quantum information science, including Miron Livny, professor of computer sciences with the Wisconsin Institute for Discovery at UW–Madison, and OpenLab of the CERN IT division. Importantly, she is also collaborating with scientists at IBM Zürich Research. Through them she has access to their quantum computer simulators and quantum computer hardware.
With IBM’s innovative quantum technologies, Wu and her team plan to overcome the challenges imposed by the large, complex datasets generated from the HL-LHC experiments. They will work on quantum feature map with entangling qubits – quantum bits used in quantum computers – to be able to encode the large datasets into the limited number of qubits. They will also work on improving quantum algorithms to advance machine learning, which they expect will lead to advances that have wide-ranging benefits outside of high energy physics.
“Although the era of efficient quantum computing may still be years away, we have made promising progress and obtained preliminary results in applying quantum machine learning to high energy physics with IBM’s resources,” Wu says. “The US government, as well as US industrial counterparts, are planning to invest heavily in quantum computing in order to lead the international competition in the area of quantum information science technology, and we are excited to be able to apply these technologies to discoveries in high energy physics.”
Wu’s DOE grant marks the second to be earned by WQI members this summer. In July, Shimon Kolowitz and collaborators were awarded a grant to identify and mitigate the sources of noise that currently limit qubit performance.
American Family Insurance and UW–Madison: Queuing up quantum computing research
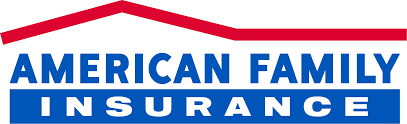
Two WQI students awarded NDSEG fellowships
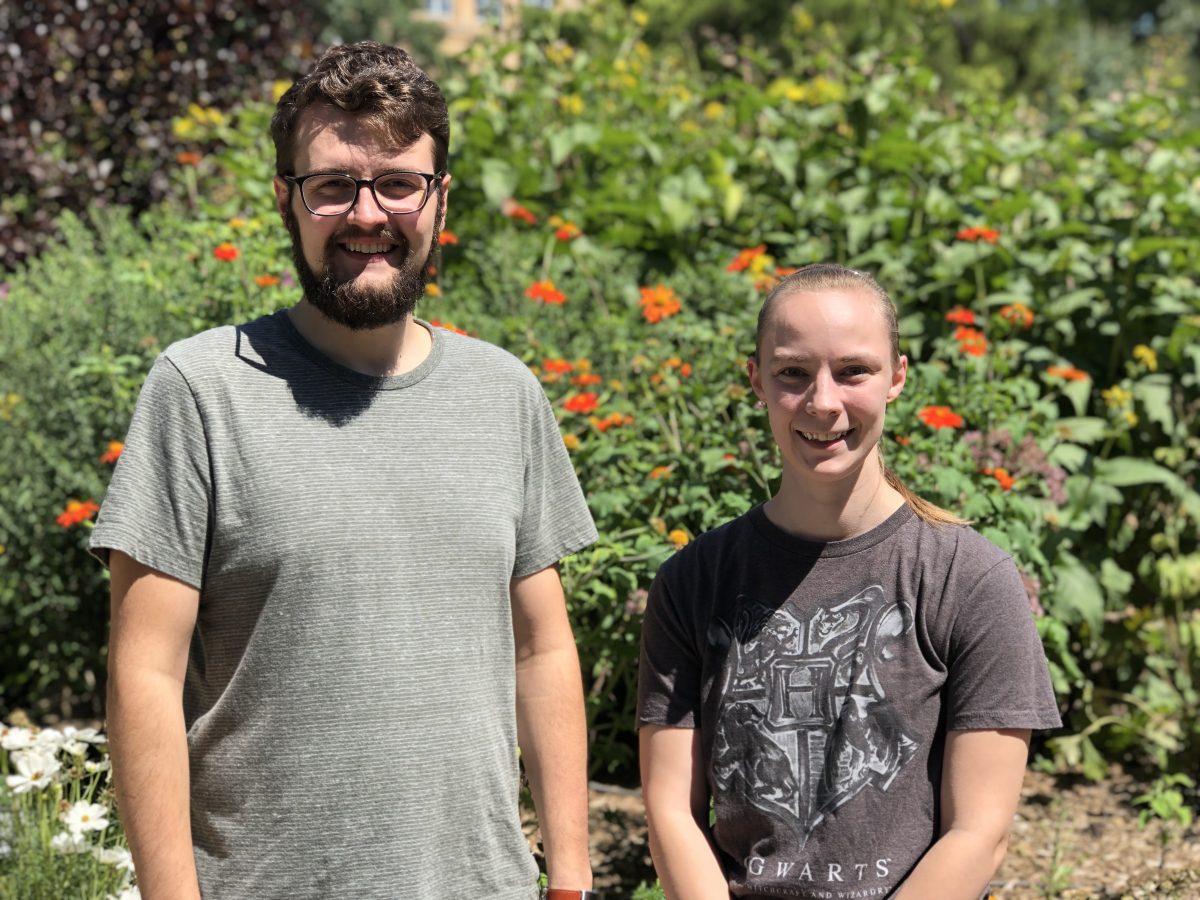
Congratulations to two WQI graduate students on earning National Defense Science and Engineering graduate fellowships!
Aedan Gardill, pictured left, and Megan Tabbutt, both second-year students in the Kolkowitz group, will receive up to three years of funding to pursue their research projects. Below, Tabbutt and Gardill summarize their research projects.
Megan Tabbutt
Optical atomic clocks are now the most precise time keepers in the world, keeping time to better than one second over the age of the universe. With support from the NDSEG, I will work with my collaborators to build a new “multiplexed” strontium optical lattice atomic clock, which will consist of two clocks in one vacuum vessel. We will use this new kind of clock to perform tests of Einstein’s theory of relativity, such as measuring the relativistic effects of gravity on the passage of time at the millimeter scale, which may one day have applications ranging from the prediction of volcanic eruptions to water resource management and flood prevention. We will also engineer strong interactions between the atoms that make up the clock to generate entangled states for quantum enhanced clock performance, among other pursuits.
Aedan Gardill
Superconducting qubits are a promising system for quantum computing, but external sources of “noise” currently limit their usefulness. A better understanding of the sources of this noise in the qubits should help advance quantum computing efforts. With the NDSEG fellowship, my research will focus on using nitrogen vacancy centers in diamond as sensors with nanometer-scale resolution. We will develop and apply novel sensing techniques to study interesting solid state systems, such as investigating the origins of noise that currently limit superconducting qubit performance.
Progress in neutral atom quantum computing with a 2D array of qubits
WQI Director Mark Saffman’s group recently published their manuscript, “Rydberg mediated entanglement in a two-dimensional neutral atom qubit array,” on arXiv.
You can access the article on the arXiv site.
Quantum computing with semiconductor spins
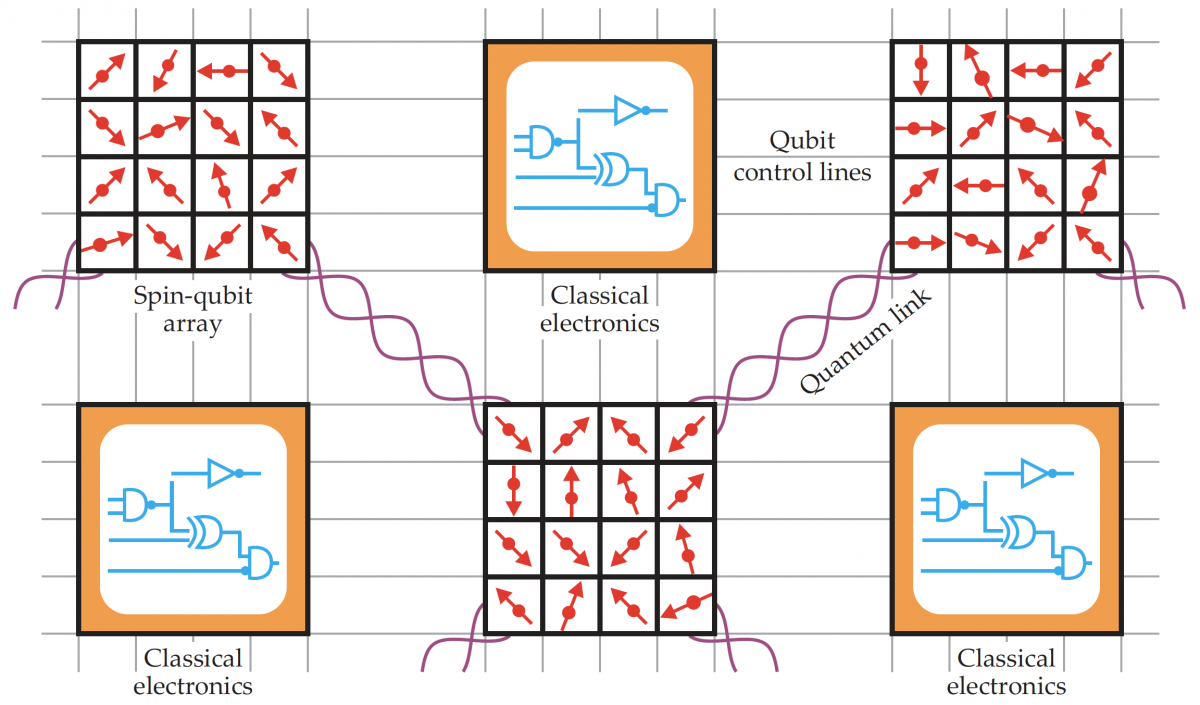
WQI’s Mark Eriksson co-authored a piece, “Quantum computing with semiconductor spins,” in the August 2019 issue of Physics Today.
The full article is available at Physics Today’s website.